Next: The NGST Mission Simulator
Up: Future Instruments and Telescopes, Tools
Previous: Future Instruments and Telescopes, Tools
Table of Contents -
Subject Index -
Author Index -
PS reprint -
Smith, E. P. 2000, in ASP Conf. Ser., Vol. 216, Astronomical Data
Analysis Software and Systems IX, eds. N. Manset, C. Veillet, D. Crabtree (San Francisco: ASP), 297
Infrared Astronomy and NGST
E. P. Smith1
NASA - Goddard Space Flight Center
Abstract:
The Next Generation Space Telescope (NGST), scheduled for launch in
2008, will be an infrared (0.6-10+

m) optimized 8m telescope,
passively cooled to 50K, located in an orbit about the second Lagrange
point (L2). NGST is a successor to the Hubble Space Telescope (HST) and
will be a key part of the NASA Origins Program. Like HST, it will be a
general user observatory and will be capable of studying a wide variety
of phenomena. For design purposes we are using a Design Reference
Mission (DRM) which contains programs investigating; the light from the
first stars and galaxies to form after the Big Bang, early supernovae
and the chemical enrichment of the universe, protostellar environments
within our own Galaxy, faint white dwarfs in Local Group galaxies,
Kuiper Belt objects (KBOs) within the Solar system to name a few. The
NGST will use many advanced technologies to realize its goals. Among
these are advanced software and control systems for the telescope and
its instruments. I give a brief update on the state of the NGST project
and projected capabilities for this observatory.
1. Science Goals
Although there have been discussions about telescopes to succeed the
Hubble Space Telescope (HST) since even before its launch, the vision
for current science goals of NGST was first articulated the HST and
Beyond report (Dressler 1996). This report outlines the enormous
potential of a scientific successor to HST, optimized for the near
infrared (NIR, 1-5
m). The goals outlined in the report have been
fleshed out and quantified by the NGST Ad Hoc Science Working Group
(ASWG) and are currently represented by the Design Reference Mission
(DRM). The DRM is comprised of a suite of observations that could be
completed in 2.5 years. Below I highlight some facets of the DRM and
the impact they would have on astronomy.
1.1. The Design Reference Mission
The goals of the DRM are to:

- Provide examples of NGST science to stimulate further
inputs from the astronomy community,

- Provide descriptions of science programs in sufficient
detail to derive secondary requirements/capabilities of the observatory,

- Provide a semi-quantitative basis for trade studies
(e.g., sensitivity versus field of view).
These science programs have been organized by the ASWG under
five themes and can be accessed through the NGST
science website2. During
the Formulation phase (Phase A/B), we will continue to solicit programs
for the DRM from our international partners and the astronomy community.
Table 1:
NGST design reference mission components
Theme Name |
DRM fraction |
|
Origin and Evolution of Galaxies |
33% |
|
Cosmology and Structure of the Universe |
21% |
|
History of the Local Universe |
16% |
|
Birth of Stars |
15% |
|
Origin and Evolution of Planetary Systems |
15% |
|
|
|
|
The Origin and Evolution of Galaxies
Figure 1:
The sensitivity of an NGST deep field (10
s in 30%
bandwidths, 10-
detection). Also indicated are the spectra of
starburst regions (10
solar masses in 10
years) and
established populations (10
solar masses at 1 Gyr) at various
redshifts (
). Comparable sensitivities also are shown
for the Hubble Deep Field using NICMOS.
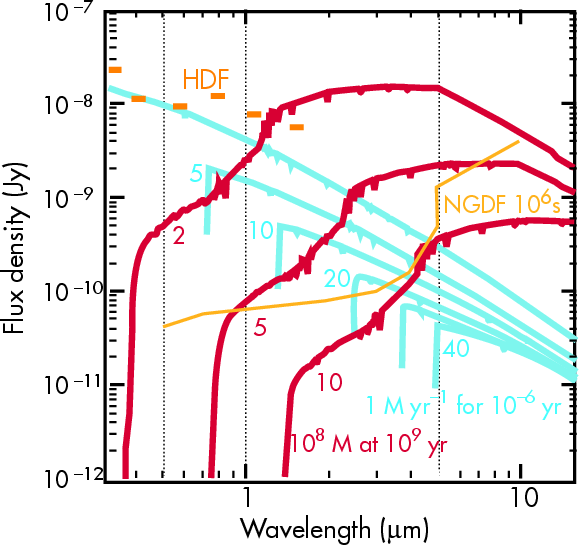 |
The primary emphasis NGST science remains on detecting and
characterizing the light from the first stars and galaxies to form after
the Big Bang. In 2008, NGST will be poised complete our understanding
of the formation and early evolution of galaxies such as the Milky Way
by:

- Detecting the earliest phases of star and galaxy
formation Ñ the end of the ``dark ages" (Figure 1). This
requires superb NIR sensitivity (
nJy, 1-4
m) in deep
broadband imaging (in a
second exposure);

- Resolving the first galactic substructures larger than
individual star clusters ( 300 pc for
). This requires
HST-like resolution in the NIR ( 0.060
at
2
m);

- Quantitatively measuring the fundamental properties of
individual galaxies. This will be enabled by emission-line and
absorption-line spectroscopy, with broad spectral coverage and
low-to-moderate spectral resolution (
):
(
m) for redshift confirmation, cluster
membership, and ages of stellar populations;
(
m or longer) for star formation rates,
metallicity, and reddening;
(
m) for dynamics (mass).

- Detecting and diagnosing dust-enshrouded regions hiding
massive star formation or active galactic nuclei during the epoch of
greatest star formation to a minimum of
.
Cosmology, Structure and Chemical Enrichment of the
Universe
The geometry and structure of the Universe, as well as its history of
element formation, is intimately related to the formation of
galaxies. The Microwave Anisotropy Probe and Planck missions will
measure the power spectrum of the Cosmic Microwave Background (CMB) at
and, using standard models, will provide or constrain key
cosmological constants. NGST will play a powerful complementary role in
determining the distribution of mass and light on small scales. Large
microlensing imaging surveys will use the wide field, superb angular
resolution, and excellent
m sensitivity of NGST to measure
the mass structure of the Universe at
on scales smaller than
those probed by CMB measurements from space or possible from the ground
or HST. Anticipated science programs
include:

- The dark matter halos of galaxies to redshifts
of
will be weighed statistically by deep imaging of selected
fields,

- The growth of galaxy clusters to redshifts of
will be measured using multi-color deep imaging of selected
high-redshift clusters and proto-clusters discovered in other surveys,

- The statistical properties of the distribution of
matter on scales of 1-10 Mpc can be found from wide-area,
high-resolution NGST imaging surveys (
deg
).
These imaging programs are comparable in depth and required
field of view to those used for the study of galaxy evolution. Such
surveys also provide an excellent method for discovering Type 1a and
Type 2 supernovae (SNe) at redshifts between
. Measuring the
rates and galactic associations of Type 1a and Type 2 supernovae will
provide an independent assessment of the history of element production.
We expect that NGST will be crucial in extending the observations of
Type 1a supernovae beyond
to
. Only at the
higher redshifts is it possible to distinguish between the behavior of
Type 1a supernovae with cosmologies involving only H
,
and
, and models with significant SNe evolution or smoothly
distributed gray obscuration. Such data will provide measurements of
the cosmological parameters, which are independent of and complementary
to those derived from the CMB missions.
The Processes of Star and Planet Formation
The potential studies in this arena are essentially limitless and depend
crucially on the available spectral resolutions and MIR wavelength
coverage. Examples include:

- Characterizing the infall and outflow processes through
which stars are built and their final masses determined. MIR
spectroscopy will diagnose the accretion shocks in protostellar systems,
while NIR imaging will reveal outflow shocks and jets near their source,
with a resolution of
2 AU;

- Tracing the structure and evolution of circumstellar
material, from the massive envelopes of Class 0 protostars to the
protoplanetary disks of pre-main sequence stars, and finally to the
dissipation of these disks into mature debris disks of main sequence
stars. NIR and MIR spectroscopy of gas and dust features, their
excitation, and their radial variation within the circumstellar region
will permit study of the growth of dust grains toward planetesimals, the
chemical processing of disk gas, and the disk dissipation mechanisms
that define the time available for planet formation;

- Detecting and characterizing substellar
objects. Ground-based sky surveys and adaptive optics programs are now
beginning to discover significant numbers of isolated and companion
brown dwarf stars. However, these observations will be limited to the
bright (high mass/low age) end of the substellar luminosity function and
to wide binary companions. NGST will have the needed combination of
high-angular resolution, high sensitivity, and a stable PSF for
high-contrast imaging of faint substellar companions in planetary
orbits. Observations at 5
m with a graded-mask coronograph will be
able to directly detect planets with Jupiter's mass, age, and orbital
semi-major axis in more than 90% of the single stars within 8pc of the
Sun (
systems). By detecting planetary photons directly, NGST
will provide the first opportunity to spectrally characterize exoplanet
atmospheres.
1.2. NGST Mission Concept
The science goals for NGST require a telescope with high sensitivity
covering the wavelength range from 0.6 to 10
m, with capability out
to 28
m, and with NIR angular resolution comparable to that of
HST. Ball Aerospace, TRW, Industry, and NASA studied three several
mission architectures during pre-Phase A
(http://www.ngst.nasa.gov/Hardware/designs.html). For simplicity,
the NASA architecture, referred to as the Reference Architecture,
is presented here. The other concepts are similar, responding to
the same high level requirements. The Reference Architecture
established the technical and financial feasibility of the mission, and
serves as a reference design to which proposed architectures and
instruments can be compared. Figure 2 shows the
observatory and its main components: the Optical Telescope Assembly
(OTA), the Integrated Science Instruments Module (ISIM) and the
Spacecraft Support Module(SSM).
Figure 2:
The reference architecture telescope design features.
 |
The Elements of the Reference Architecture Concept
The Reference Architecture optical configuration is a three-mirror
anastigmat that provides a real, accessible pupil and permits
a-relatively fast primary mirror to minimize telescope length. This
design provides excellent imaging over a field of more than 20 arcminutes
with achievable alignment tolerances. A real pupil permits the use of a
deformable mirror (DM) for wavefront correction, and a-fast-steering
mirror for fine pointing using image compensation. The primary mirror
is a compact 8 m diameter segmented aperture. It is composed of a
central mirror segment, with a diameter of 3.3 meters, surrounded by
eight petals. The petals are folded alternately up and down and
deployed after launch. The Reference Architecture mirror is made of
beryllium, thermally controlled with very low power heaters (20 mW total)
so that its figure remains insensitive to rapid or large positioning
slews. The areal density of the primary mirror assembly (mirror,
actuators and backup structure) is 13 kg m
. The DM provides a
design margin for figure errors in the primary mirror,including those
due to gravity release, thermal gradients, or edge effects. The DM will
correct the wavefront so that the system will be diffraction limited
below 2
m. Unlike telescopes such as HST that are launched fully
assembled, NGST must be able to compensate for errors in deployment
position, long-term dimensional changes, and on-orbit thermal variations.
Optics are aligned and phased by observing the image of a star and
deriving mirror position corrections. Wavefront errors are determined
by obtaining defocused star images and analyzing the image with a
``phase retrieval" computer algorithm (Redding et al. 1998).
Simulation of typical wavefront errors due to polishing, thermal
gradients, etc., and diffraction effects due to aperture notches, gaps
and obstruction of the
secondary mirror support, indicate that the final image will have a
Strehl ratio of about 81% at 2
m, and 60% at 0.6
m without
additional DM correction.The Integrated Science Instrument Module (ISIM)
consists of a cryogenic instrument module integrated with the OTA, and
processors,software, and other electronics located in the Spacecraft
Support Module. The ISIM provides the structure, environment, and
data handling for several modular science instruments as well as
components of the OTA system - the tertiary mirror, DM, and
fast-steering mirror.The following ISIM design is illustrative and is
not intended to define NGST's final complement of instruments. A wide
range of pre-Formulation phase (pre-Phase A) studies of ISIM
architecture and individual science instruments have been conducted by
science community teams in the US, Europe, and Canada. Procurement
responsibility for the science instruments will be allocated among NASA,
ESA, and CSA during the Formulation phase (Phase A/B), and instrument
proposals solicited by those agencies following selection of the flight
NGST architecture. The Reference Architecture instrument suite
includes:

- A NIR camera covering 0.6 to 5
m, critically sampled at 2
m. Efficient surveying capability,
as well as guiding requirements, set the field at about 4
x
4
,apportioned over four sub cameras each covering a field of
2
x2
. The NIR detectors (InSb or thinned HgCdTe) are
radiatively cooled to 30 K;

- A NIR multi-object
spectrometer, with spectral resolutions of 300 and 3000 and a spatial
resolution of 100 mas, covering a field of 3
x 3
.
Multi-object capability is enabled by an array of
2048
micro-mirrors used to form a reflective slit mask, directing
light into or away from the spectrometer;

- A MIR
camera/spectrometer covering a field of 2
x 2
with
a-spectral range of
m using a 1K x 1K Si:As array as
detector,and a long slit cross-dispersed grism. Its spectral
resolution is
. The camera employs a selection of slits and
a no-slit option to enable direct imaging with filters. The MIR
detector is cooled to 6 Kby a miniaturized reverse turbo-Brayton
cooler; open cycle solid hydrogen cooling has been identified as a
viable alternative.
Following the 1996 NGST study, the NASA
Project undertook a detailed design study of the ISIM to demonstrate
engineering feasibility of the mission's science goals, assess the
required technologies, and revisit the cost estimates. This study
concluded that all engineering requirements of the baseline
instrument complement including detector, thermal, and data system
requirements are feasible with technology that is expected to be mature
in 2003 at the beginning of the Implementation phase (Phase
C/D).
2. Project Update
The NGST is being
developed by a broad consortium of government laboratories, academic
institutions and aerospace industry partners. Each of these groups
bring unique strengths to the project. This past year saw the selection
of the Phase A industry partners; Lockheed-Martin and a consortium of
Ball Aerospace and TRW. Each of these two teams will develop
independent architectures for the observatory working in conjunction
with NASA, STScI and the international partners. Support for NGST is
strong at NASA headquarters and the funding remains stable for the
ensuing fiscal year. Twelve ISIM studies by US and international teams
have been completed. These studies will be used to assist the project
scientist in recommending a suite of capabilities that NGST
requires. Once this suite is defined NASA, ESA and CSA will negotiate
the allocation of responsibilities.
3. Operations Considerations
One distinct advantage we have in
building NGST is that the operating organization, the Space Telescope
Science Institute (STScI), was selected early on in the project's
history. By having the operations institution participate in the early
decisions about the configuration of the observatory and its
instruments we can optimize the system trades and costs. From its
conception NGST was envisioned as an observatory that would be simpler
to operate than HST. The primary simplification comes from its distant
orbit and corresponding lack of Earth occultations and the
complications that these induce. Advances in computer hardware and
software will also allow NGST to operate in a more ``event-driven''
mode rather than a strict time line manner like HST or other low Earth
orbit missions. One could even imagine making use of the vast
ground-based data archives that will exist by the launch of NGST to
provide lists of alternate ``interesting'' targets near any given
scheduled target which the observatory could slew to in the event that
the primary acquisition fails. Such alternate targets could be
autonomously selected when the next program in the event queue could
not me started for engineering reasons (e.g., momentum management, sun
angle constraints).Since the final instrument suite for NGST is not yet
known we cannot determine just how the notion of operational simplicity
will reflected in their design. STScI has begun to study a number of
issues related to observatory operability and their associated impacts
on the achievable science and mission costs. These include: proposed
dithering strategy, observation overheads, sun shield imposed
restrictions, guiding methodology, ground segment, on-board data
processing.
4. Discovery Potential
NGST will
be an observatory with enormous discovery potential both at 0.6-10
m and at longer wavelengths. This is illustrated in Figure 3,
which shows the time to achieve a broad-band, high-resolution wide
field image with a variety of facilities: NGST, HST, Gemini
(representing IR-optimized ground-based 8 m telescopes), and Space
Infrared Telescope Facility (SIRTF). NGST enjoys a significant
background advantage over the ground at all wavelengths, a larger field
of view over which high-resolution images can be obtained
(
assumed) and a significant aperture advantage
over SIRTF. The shorter times required to achieve a given threshold
sensitivity can translate into larger fields observed (more targets) or
greater
sensitivities.
Figure 3:
NGST
Discovery Space: the relative speed of broadband NGST high resolution,
wide field imaging compared with other observatories (HST, Gemini, and
SIRTF).
 |
For wavelengths between 2.2 and 10
m, NGST is many orders of magnitude faster than any other planned
facility. In practice, it will have sensitivities several orders of
magnitude better than even SIRTF because of source confusion in very
deep observations. In this regard, SIRTF is a superb instrument for
surveys of 10-100 square degrees. NGST is best used for deeper
observations of smaller pre-selected fields. At wavelengths longer
than 10
m, NGST will surpass SIRTF by an order of magnitude in
sensitivity despite a much higher MIR background. For NIR and MIR
spectroscopy, it will be unsurpassed between 0.9 and 28
m (28
m is the cutoff for Si:As detectors) because NGST's thermal
background is much reduced at high spatial resolution. The ``uniqueness
space" for NGST is defined relative to the many large ground-based
telescopes that will be operating by 2008. The criterion is that NGST
be at least 100 times faster than an optimally operated and equipped 8 m
telescope with laser guide stars and adaptive optics. In the NIR, the
NGST imaging uniqueness space is very large. For moderate spectral
resolution 2-D spectroscopy the effects of detector noise significantly
narrow the uniqueness space. At this resolution and detector dark
current,
0.02 e
pixel
s
, NGST is no longer
background limited in the visible or NIR (
m). For spectroscopy
of single faint targets, for instance, NGST would be used primarily in
the NIR (
m). For high-resolution spectroscopy in the visible
and J and K bands (
) or for visible imaging of large fields
with 0.4
resolution, large ground-based telescopes are
competitive with NGST. This is where the large telescopes planned for
the next decade will make major contributions.It is instructive to
consider the relative power of NGST compared with that of HST. HST is
currently unique in the windowed ultraviolet(0.1-0.3
m, a factor
of three in wavelength). It has comparable sensitivity but superior
resolution over ground-based telescopes in the visible and NIR (0.3-1.8
m, a factor of 6). NGST will have10 to 100 times more imaging
sensitivity and superior resolution at 2.5
m (a factor of 4,
i.e. comparable to HST at 0.6
m). It will be unique in imaging and
spectroscopy from 2.5-28
m (a factor of 10 in wavelength). It is
clear from this simple analysis that NGST will have at least as great an
impact on astronomy as HST.
Acknowledgments
I wish to thank John Mather,
Patricia Pengra, Peter Stockman and the NGST team for providing
graphics and input used in this
presentation.
References
Dressler, A. 1996, HST and Beyond, (AURA:
Washington)
Redding, D. et al. 1998, SPIE, 3356, 47
Footnotes
- ... Smith1
- Code 681, Laboratory for Astronomy and Solar Physics
- ... website2
- http://www.ngst.nasa.gov/science/
© Copyright 2000 Astronomical Society of the Pacific, 390 Ashton Avenue, San Francisco, California 94112, USA
Next: The NGST Mission Simulator
Up: Future Instruments and Telescopes, Tools
Previous: Future Instruments and Telescopes, Tools
Table of Contents -
Subject Index -
Author Index -
PS reprint -
adass@cfht.hawaii.edu